LENS
Core Mystery
What we know—and don't—about what's at the center of the Earth
We know more about the surfaces of other planets than we do about what's inside our own. No drill has ever penetrated Earth's crust, which averages 25 miles thick beneath the continents.
So, virtually everything geologists know about Earth's deep structure is from seismographs, which record how seismic waves from earthquakes travel throughout the planet. "It's as if the only way of learning about the interior of the human body was through imagery such as X-rays or MRIs, with no direct access such as by inserting a probe or performing surgery," said James Van Orman, PhD, a professor and chair of the Department of Earth, Environmental and Planetary Sciences at Case Western Reserve.
During his career, Van Orman has tried to understand how Earth's core formed. Then about two years ago, Van Orman and three Case Western Reserve colleagues gradually realized there was a major overlooked obstacle to the most widely accepted geological theories. So in February, they published a study in Earth and Planetary Science Letters giving other geologists something to think about.
All seismographic evidence indicates Earth—about 8,000 miles across—is layered like an onion. Its relatively thin crust rests on an 1,800-mile-thick layer of solid rock under such heat and pressure that it slowly deforms like an extremely viscous liquid (visualize hot asphalt under a steam roller). Deeper still is the Earth's hot, liquid iron-nickel outer core, larger than the moon.
Like a peach pit, at the very center is the inner core, some 1,500 miles across. The ways seismic waves reflect from, bend (refract) around or cast shadows reveal this inner core to be purer, solid iron. "Not just solid, but crystalline," said Van Orman, meaning the iron atoms have a regular, geometric internal structure.
Problem is, Van Orman said, no geologist or metallurgist can figure out how molten iron at the unimaginable densities at Earth's very center ever started to crystallize about a billion years ago. And this conundrum is challenging fundamental geological theories about how Earth formed.
Once started, crystallization spreads rapidly. However, forming the first crystal is always tough: The first atoms in a cooled liquid require extra energy to arrange themselves in the needed geometric structure. Until the study by Van Orman and his colleagues, geological models have overlooked this extra energy—which metallurgists call the "nucleation barrier."
At ordinary temperatures and pressures, nature finds that extra energy in two different ways, Van Orman explained. First, some microscopic impurity such as a grain of dust or a tiny bubble can offer a "nucleation surface" where crystallization can begin. However, Van Orman noted, "it's hard to imagine how a bubble or grain of dust could be transported to the center of the core" and then survive Earth's hellish inner core, which is hotter than the surface of the sun and has pressure thousands of times more crushing than in the deepest ocean trench.
Alternatively, a liquid without such impurities can "supercool" significantly below its normal freezing temperature until crystals spontaneously form. But the Van Orman team calculations reveal that Earth's inner core would need to supercool by an impossible 1,800 degrees Fahrenheit.
Because neither way nature normally finds the missing nucleation energy seems possible at the extreme temperatures and pressure inside the inner core, Van Orman has no solution to the big mystery he and his co-authors have identified: how iron could crystallize there. But seismological evidence is undeniable: Crystalline iron exists at the center of Earth.
"Our least crazy idea we threw out to the scientific community in February," Van Orman said, referring to the study. The co-authors calculated that roughly mountain-sized chunks of iron released from the bottom of the viscous mantle could survive sinking through the molten outer core into the inner core to become nucleation sites. "We'll see how other scholars respond."
Earth's Layers
- The crust is ~25 miles thick under the continents.
- The mantle is ~1,800 miles thick and is composed of viscous solid rock.
- The outer core is ~1,400 miles thick and is composed of liquid iron-nickel alloy.
- The solid inner core has a radius of ~760 miles and is composed of nearly pure crystalline iron-nickel.
- The deepest oil rig has drilled only 40,502 feet (~7.6 miles)—about the distance underground that intercontinental jets fly overhead.
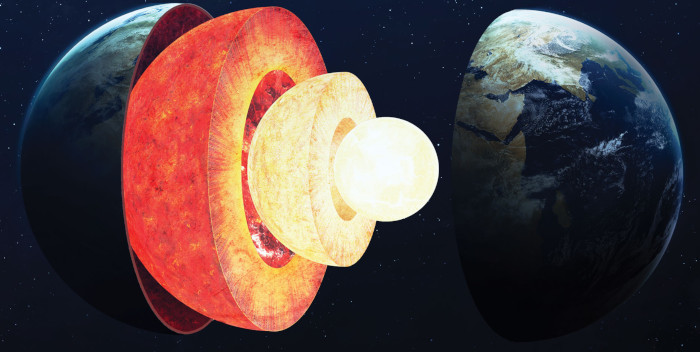
Extreme Conditions
At 11,000°F, the Earth's inner core is similar in temperature to the surface of the sun.
At Earth's center, the pressure is 3.6 million times atmospheric pressure—3,350 times more crushing than in the deepest part of the Marianas Trench.
The Puzzling Mystery of What Happened to Iron at Earth's Core
Forming the first crystal of a crystalline solid requires extra energy for disordered atoms in a liquid to arrange themselves in ordered geometry. So, how did Earth's inner core start to crystallize?
Could mountain-sized chunks of iron released from the bottom of the mantle have drifted through the outer core to the inner core, triggering crystallization?
WHAT WE CAN LEARN FROM SEISMOLOGY
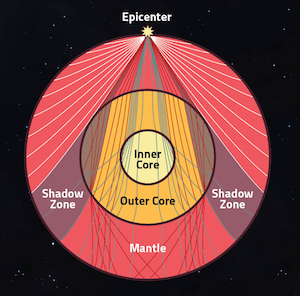
The main way geologists know about the structure of Earth's interior is by studying the patterns and arrival times of vibrations—known as seismic waves—that emanate from large earthquakes and travel through Earth's interior. For example, one type, known as P-waves, can bounce off the inner core and veer off their expected course, arriving in an area known as "the shadow zone." These waves provided the first evidence for the existence of a solid inner core.
P-wave passing through mantle
P-wave passing through mantle and liquid outer core
P-wave interacting with solid inner core