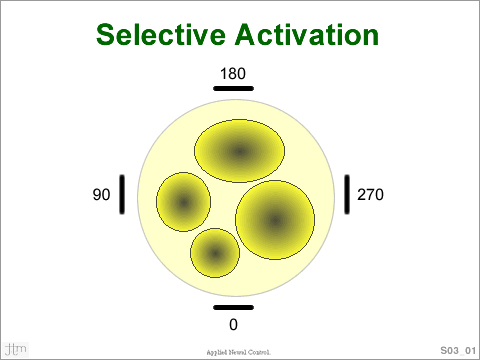
Electrical stimulation of nerves usually activates all the
axons within the influence of the electrical field. To achieve
functional goals, it is necessary to be able to stimulate
select subpopulations of axons directed towards specific
targets. The nervous system evolved mechanisms to signal
specific locations with specific neurotransmitters. The individual
axons distribute these signals spatially to achieve desired
function. Electrical intervention to establish the same capacity
needs to be able to provide signals in a similar pattern.
This requires the ability to selectively activate sets of
axons within a peripheral nerve. Advantages may be taken
of gross anatomical paths, fascicle distribution within nerves,
differences in axon diameters or utilize reflex pathways.
The applied electrical field can be varied temporally to
manipulate the non-linear properties of the axonic ion-channels
to generate required firing patterns.
The creation of technology to selectively activate specific
populations of neurons
and electronically reposition “virtual excitation sites” will bring
neural prostheses that have tunable electrodes. Very often the site of injection
of applied current, the electrode contact, of an implanted electrode is not at
the best location to electrically activate a desired population of neurons. With
a tunable electrode, we would be able to create a “virtual electrode site” at
a desired position without physically moving the electrode.
There are at least four* phenomena that we can draw upon to create these electronically
steerable electrodes:
1) The electrically induced potential is inversely related to the separation
between the site of current injection and the point of interest,
2) Current injected from separate contacts generate electrical potentials that
are linearly additive,
3) Voltage-gated sodium ion channels are not uniformly distributed over the surface
of the neuron; and
4) Voltage-gated sodium ion channels exhibit an inactivatable state.
The fact that the potential falls off inversely with distance means that the
effects of an applied current are more pronounced on neurons that are closer
to the contact site than those that are further way from the current injection
site; usually this is taken to mean that neurons closer to the electrode require
lower currents to activate, but as we will discover there is more to this statement.
The fact that voltage-gated sodium ion channels are concentrated in specific
regions of a neuron, the axon hillock and the nodes of Ranviér, means
that potential changes only in those regions can effect propagated action potentials.
The fact that voltage-gated sodium ion channels can be put into an inactivatable
state open opportunities to alter the excitability of neurons close to the contact.
Using these phenomena we will show how the normal recruitment order, large diameter
to small diameter axons, can be reversed, currents from several electrodes can
be used to “field steer” an excitation site, and the inactivation
property can enable us to effect excitation of neurons further from a current
injection site without exciting neurons closer to the electrode.
* The reader is encouraged to think of other phenomena that might be useful in
creating steerable “virtual electrode sites”.
|